Introduction to super resolution microscopy
The diffraction limit defined by Abbe (1873) corresponds to the radius of the spot where the light is diffracted. The Abbe diffraction limit depends on the light wavelength (λ), the refractive index of the medium (n) and the half-angle of the converging spot (ϴ). One limiting parameter is the numerical aperture (NA) and nowadays best optics reach about 1.4 NA leading to an Abbe limit of λ/2NA i.e. 0.25µm (for green light at 500nm) (200nm laterally/ 500nm axially). This is much higher than the resolution needed to observe and discriminate between different single molecules or compounds inside a cell. Super-Resolution microscopy (SRM) allows scientists to pass the diffraction limit of light, giving them the chance to observe cellular structures at the nanometer scale[1], from entire organelles to individual proteins.
The Super-Resolution microscopy techniques can be mainly classified in two groups that differ in lateral/axial resolution and in their inherent maximal temporal resolution. First are the patterned light illumination techniques such as Stimulated Emission Depletion (STED) and Structured Illumination Microscopy (SIM). Second are localization-based techniques which includes Stochastic Optical Reconstruction Microscopy (STORM) and Photo-activation Localization Microscopy (PALM).
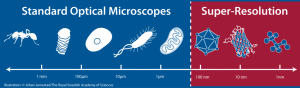
What is Super-Resolution Structured Illumination Microscopy (SR-SIM)?
The Super-Resolution SIM technique principle is to use interference-generated light patterns to create a Moiré effect. This allows to extract information with higher resolution. The Moiré effect consists in the superposition of two periodic structures that produce a down-modulation of the frequencies. For example, two structures of high spatial frequency can produce a low spatial frequency when they overlap. Using this configuration only zero order and/or first order diffracted laser beams, depending if the goal is to achieve 2D or 3D Super-Resolution Structured Illumination microscopy, are let to pass through the objective. The laser beams interfere between them at the focal plane of the objective and create an illumination in stripes. The intensity is hence following a sinusoidal wave.
If the illumination is homogeneous (normal illumination without using any striped pattern) the point-spread-function of the objective makes not possible to distinguish the two objects being separated by a small distance (the real distance) which is bigger than 200nm.
Using a structured illumination, the combination between the high frequency organization of the objects within the sample and the high frequency of the illumination stripes creates a low frequency pattern, allowing to distinguish two objects that are separated by 200nm.
Finally, to reconstruct the final super-resolved image (Super-Resolution image), several images need to be collected. Each of them need to be acquired using different orientations of the structured illumination. All this is done by moving the diffraction grating (translation and rotation). For example, to get a 2D-Sr-sim image, it is necessary to have nine raw images, with three translation and three rotations. To get a 3D one, 15 images are necessary, using 5 translations and 3 rotations.
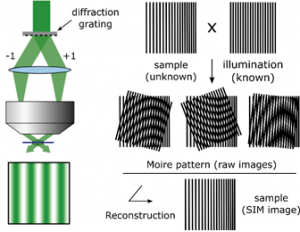
Ultra fast temperature shift device for in vitro experiments under microscopy
Strenths and weaknesses of the super-resolution SIM
Super-Resolution SIM increases by two the spatial resolution over wide-field microscopy. In 2D/3D-SIM, lateral and axial resolutions are improved by 100nm and 250nm respectively.
Advantages
SR-SIM also allows to obtain 4D images at fast frame rate. Normally, it is necessary to have nine to filthen raw images for 2D/3D-SIM, while for STORM/PALM it is necessary to have at least one thousand.
The use of conventional fluorophores is possible giving access to existing biological mutated cell lines.
Up to four different wavelength[1] can be used with Structure Illumination microscopy making this technique very useful to co-localize or access to multiple highly-resolved information inside cells and organelles.
Finally, the sample preparation for SR-SIM observations is easier than other super-resolution techniques such as STED or STLM[2].
Limitations
This super resolution microscopy technique is very sensitive to out-of-focus light and it is very hard to focus in samples that are thick or those which are densely labelled[3].
In addition, whereas PALM/STORM or STED techniques can reach a 20nm lateral diffraction limit, Structured-illumination microscopy is limited to 200nm[4].
Finally, some artefacts may be generated during the image reconstruction which can damage the quality of the image [5].
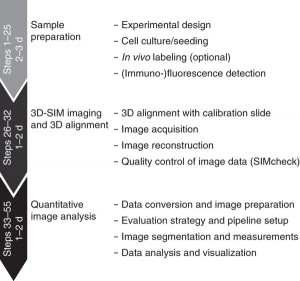
References
[1] Sydor AM, Czymmek KJ, Puchner EM, Mennella V. Super-Resolution Microscopy: From Single Molecules to Supramolecular Assemblies. Trends Cell Biol [Internet]. 2015 [cited 2017 Jun 15];25:730–48. Available from: http://www.cell.com/trends/cell-biology/pdf/S0962-8924(15)00191-9.pdf
[2] Wegel E, Göhler A, Lagerholm BC, Wainman A, Uphoff S, Kaufmann R, et al. Imaging cellular structures in super-resolution with SIM, STED and Localisation Microscopy: A practical comparison. Sci Rep [Internet]. 2016;6(1):27290. Available from: http://www.nature.com/articles/srep27290
[3] Jost A, Heintzmann R. Superresolution Multidimensional Imaging with Structured Illumination Microscopy. Annu Rev Mater Res [Internet]. 2013 Jul [cited 2017 Jun 16];43(1):261–82. Available from: http://www.annualreviews.org/doi/10.1146/annurev-matsci-071312-121648
[4] Henriques R, Mhlanga MM. PALM and STORM: What hides beyond the Rayleigh limit? Biotechnol J [Internet]. 2009 Jun [cited 2017 Jun 21];4(6):846–57. Available from: http://doi.wiley.com/10.1002/biot.200900024
[5] Kraus F, Miron E, Demmerle J, Chitiashvili T, Budco A, Alle Q, et al. Quantitative 3D structured illumination microscopy of nuclear structures. Nat Protoc [Internet]. 2017;12(5):1011–28. Available from: http://www.nature.com/doifinder/10.1038/nprot.2017.020