Brain on a chip proof of concepts: what for?
Organs-on-chips are promising biological on chip laboratories expected to allow robust and accurate drug testing. Whether these micro-devices will be an alternative solution to animal drug testing, a revolutionary approach for personalized medicine or a complementary resource in the drug development industry remains to be addressed. Nevertheless, several laboratory proof-of-concept have been successfully performed, demonstrating the relevance of this approach. In this mini-review, we focused on devices mimicking the blood-brain barrier. These “Brain on chips” combine both the in vivo and in vitro advantages given that mimicking the reality and developing a simple ex vivo model is well balanced.
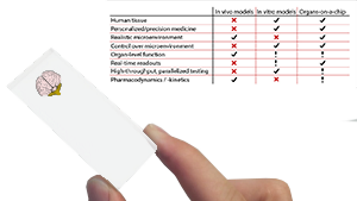
How to culture vascularized & immunocompetent 3D models in a standard Multiwell
Brain on-chip: focus on the blood-brain barrier
Within the neurovascular unit (NVU), the blood brain barrier (BBB) is composed of specialized endothelial cells that separate the brain tissues from the blood system (Fig. 2a). In order to target specific areas of the brain, drugs traveling through the blood vessels must cross this barrier. Studying the BBB is therefore key to understand brain drug targeting and efficiency.
In vivo techniques are still the best way to study the BBB. They reflect the full BBB complex environment and its diversity. However, 80% of drugs successfully tested on animals fail the following clinical testing. This points out the necessity to develop alternative solutions to costly, time-consuming, and ethically contentious animal drug testing. On the other hand, in vitro Petri dishes solutions, though offering an easy model suitable for large-scale drug testing, do not reflect the complexity of the BBB and are not suitable to address complex scientific questions.
To date, few “brain-on-chip” solutions reflecting the BBB system have been published. Van der Helm and colleagues reviewed these solutions in a paper published in Tissue Barrier in 2016 [1].
- Booth and Kim (2012 [2], fig. 2b-I): a micro-BBB model. This double-layer PDMS micro-device integrates a polycarbonate (PC) membrane, sandwiched in two glass slides. A 4-point metallic electrode deposition (a key standard in transendothelial electrical resistance (TEER) measurement) allowed the author to characterize the relevance of their system.
- Yeon et al. (2012 [3], fig. 2b-II): a permeability assay system for cerebral microvasculature. This PDMS-made device allows generating two different flows in two adjacent micro-channels leading to a pressure difference. This allowed us to trap HUVECs cells and generate a suitable barrier after 23h to test various drugs.
- Griep et al. (2013 [4], fig. 2b-III): a BBB-on-a-chip. This PDMS based microdevice consists of two microchannels separated by a PC membrane. The top channel (coated with collagen I) hosts hCMEC/D3 cells and a barrier is formed at the membrane after 2 days.
- Achyuta et al. (2013 [5], fig. 2b-IV): a quasi-NVU-on-a-chip. Interestingly, this PDMS-based device is composed of two independent parts that can be used separately for cell culture. Once assembled, it emulates a fairly complex NVU environment allowing advanced molecule trafficking studies across the BBB.
- Prabhakarpandian et al. (2013 [6], fig. 2b-V): a SyM-BBB device. This PDMS-on glass system introduced the ability to simultaneously image the brain tissue compartment and the blood compartment. In this case, the communication was designed with micro-perforations in the PDMS itself.
- Cho et al. (2015 [7], fig. 2b-VI): a 3-dimensional BBB-on-a-chip. Poly-D-lysine and collagen were used to host RBE4 rat endothelial cells allowing a barrier to be formed by two thin layers of cells at both sides of the membrane. Neutrophil migration through this barrier was then monitored with or without the addition of a chemoattractant (interleukin 8).
- Kim et al. (2015 [8], fig. 2b-VII): a collagen-based 3D model of brain vasculature. Mouse bEnd.3 endothelial cells were cultured in 3D printed micro-tubes (a pattern including fluidic connector drills) to mimic the BBB. Fluorescence images tracking labeled dextran proved the functionality of the system and the barrier’s permeability. Mannitol assays further characterized the permeability and its recovery over time. Blood brain barrier microfluidic models
- Brown et al. (2015 [9], fig. 2b-VIII): a full NVU-on-a-chip. This more complex PDMS-based device is composed of three layers respectively for a vascularization chamber separated from a brain chamber by a PC membrane and a third brain perfusion layer with micro-channels. As in the previous device, tests with FITC-labeled dextran could characterize the permeability of the BBB. Worthwhile to mention, the authors used induced pluripotent cells stem cells (hiPSC)-derived neurons. The TEER was also measured using a 4-point electrode system and a 33°C cold-shock induced a significant decreased in TEER.
- Sellgren et al. (2015 [10], fig. 2b-IX): a microfluidic NVU model. Two PDMS layers were separated with channel imprints with either a PTFE or PC membrane. Astrocytes in a collagen hydrogel and then bEnd.3 cells in collagen IV-fibronectin (PTFE) or collagen I (PC) were then cultured in the device. Thanks to the entire transparency of the device, phase contrast microscopy could be used as an interesting readout of the system. After removing the astrocytes, the authors characterized the endothelial barrier with similar techniques (FITC-dextran). Shear stress of cells was nicely-investigated using immunofluorescence to follow claudin-5 gene expression. This study showed that a PTFE membrane was more suitable than a PC one for cell attachment under relevant shear stress.
- Walter et al. (2016 [11], fig. 2b-X): a barrier-on-a-chip microfluidic system. The two authors also used this hardware design for intestinal and lung epithelial barriers. Two PDMS layers separated by a PET membrane (sealed with silicone) were sandwiched in between two glass slides. Electrodes in a 4-point sensing structure allowed TEER measurements. PDMS-based reservoirs were also integrated on the top glass slide. Either hCMEC/D3 cells or primary rat endothelial cells (co-cultured with astrocytes and pericytes) were used as cell models. Again, TEER assays and FITC-dextran/Evans-blue albumin tracking allowed the authors to characterize the barrier permeability. Confocal microscopy could also be used.
BBBs-on-chips characterization: permeability
All the studies mentioned above pass through characterization steps in order to validate the on-chip system. The first key parameter is the effective permeability of the barrier. Is it permeable? Is this permeability relevant compared to physiology? Drug testing cannot be achieved without a physiologically relevant permeability of the blood-brain-barrier model. A general view of the barrier selectivity is given in Table 1. Standard Transwell systems are well-known systems for cell-migration assays and are commonly used for barrier assays, thus they represent an interesting standard for permeability characterization. However, these static systems have an inherent drawback as solutes accumulate due to the absence of flow rate. Organs-on-chips offer an interesting advantage over these systems first as perfusion systems can be used and second as it is possible to dynamically observe the permeability in real-time using live-microscopy techniques. However, the flowrates are generated by active pumping (pressure generators, peristaltic pumps…) which could lead to a bias in permeability. One has to take this parameter into account and use ad hoc membranes with perfectly controlled flow rates (such as those generated by dedicated microfluidic pressure controllers).
BBBs-on-chips characterization: TEER
Transendothelial electrical resistance (TEER) is a parameter assessing the barrier’s tightness. The tighter is the barrier (tight cell-cell junction), the smaller will be the transport of charged molecules resulting in high resistance of the barrier. The 4-point metallic electrode measurement system measures the conductivity of the system and therefore measures its resistance (inversely proportional) and consequent tightness of the junctions. In microfluidic devices, large surface/volume ratios can strongly affect this parameter so one has to cautiously design the electrode deposition inadequate channels where the resistance scales oppositely to the cross-sectional area and the dimensions (micro) lead to high inherent electrical resistance. Though TEER techniques are non-invasive and label-free, it has to be handled and analyzed carefully for comparative studies.
BBBs-on-chips characterization: cells
The expectation that organs-on-chips will be powerful alternatives to animal drug testing requires the use of human cell models. Endothelial cells derived from brain capillaries have the appropriate expression profile BUT this phenotype is lost in vitro over time. Brain-derived endothelial cell lines are more readily available and provide less batch-to-batch difference BUT are challenging to get and also lose their phenotype over time. Brain-specific endothelial cells from human induced pluripotent stem cells (hiPSC) are of great interest in personalized medicine and already show promising results. In addition to endothelial cells, astrocytes and pericytes are key to form and maintain the barrier in the NVU context. This notably affects tight junctions and BBB features. The thinner would the membrane be, the more physiologically relevant would be the final barrier as it would allow a direct cell-cell contact. Here, membrane engineering is an important aspect to allow ultra-thin and biocompatible membrane manufacturing.
BBBs-on-chips characterization: shear stress
Shear stress is important for endothelial differentiation and function. Unlike static in vitro models (Transwell), microfluidics provides a powerful way of mimicking such shear stress by enabling highly-controlled flow rates. As mentioned earlier, dedicated, highly stable, and precise flow controllers coupled to relevant micro-channel designs are therefore important to ensure proper shear stress and a physiologically relevant model.
Conclusion
A blood-brain barrier on a chip (BBB-on-a-chip) is a powerful tool that emulates the real human physiology given that important parameters are controlled. Among them, van der Helm and colleagues [1] identified the permeability of the barrier (need for permeability coefficients is outlined), its tightness (through TEER measurements and standardization), the use of adequate cell types (e.g. hiPSC), and the proper design of the hardware microfluidic chip coupled to high-precision instruments for perfect environmental control of the biological tissues.
References
[1] Van der Helm MW, van der Meer AD, Eijkel JCT, van den Berg A, Segerink LI. Microfluidic organ-on-chip technology for blood-brain barrier research. Tissue Barriers. 2016; 4(1):e1142493. doi:10.1080/21688370.2016.1142493.
[2] Booth R, Kim H. Characterization of a microfluidic in vitro model of the blood-brain barrier (mu BBB). Lab Chip 2012; 12:1784-92; PMID:22422217; http://pubs.rsc.org/en/Content/ArticleLanding/2012/LC/c2lc40094d#!divAbstract
[3] Yeon JH, Na D, Choi K, Ryu SW, Choi C, Park JK. Reliable permeability assay system in a microfluidic device mimicking cerebral vasculatures. Biomedical Microdevices; 2012; 14:1141-1148, figure 1b; PMID:22821236
[4] Griep LM, Wolbers F, de Wagenaar B, ter Braak PM, Weksler BB, Romero IA, Couraud PO, Vermes I, van der Meer AD, van den Berg A. BBB ON CHIP: microfluidic platform to mechanically and biochemically modulate blood- brain barrier function. Biomedical Microdevices; 2013; 15:145-150, figure 1a and 1c; PMID:22955726
[5] Achyuta AKH, Conway AJ, Crouse RB, Bannister EC, Lee RN, Katnik CP, Behensky AA, Cuevas J, Sundaram SS. A modular approach to create a neurovascular unit-on-a-chip. Lab Chip 2013; 13:542-53; PMID:23108480; http://dx.doi.org/ 10.1039/C2LC41033H
[6] Prabhakarpandian B, Shen MC,Nich- ols JB,Mills IR, Sidoryk-Wegrzynowicz M, Aschner M, Pant K. SyM-BBB: a microfluidic blood brain barrier model. Lab Chip 2013; 13:1093-101; PMID:23344641; http://dx.doi.org/10.1039/c2lc41208j
[7] Cho H, Seo JH, Wong KH, Terasaki Y, Park J, Bong K, Arai K, Lo EH, Irimia D. Three-Dimensional Blood-Brain Barrier Model for in vitro Studies of neurovascular pathology. Sci Rep 2015; 5:15222
[8] Kim JA, Kim HN, Im SK, Chung S, Kang JY, Choi N. Collagen-based brain microvasculature model in vitro using three- dimensional printed template. Biomicrofluidics 2015; 9:024115.
[9] Brown JA, Pensabene V, Markov DA, Allwardt V, Neely MD, Shi M, Britt CM, Hoilett OS, Yang Q, Brewer BM, et al. Recreat- ing blood-brain barrier physiology and structure on chip: A novel neurovascular microfluidic bioreactor. Biomicrofluidics 2015; 9:054124.
[10] Sellgren KL, Hawkins BT, Grego S. An optically transparent membrane sup- ports shear stress studies in a three-dimensional micro- fluidic neurovascular unit model. Biomicrofluidics 2015; 9:061102.
[11] Walter FR, Valkai S, Kincses A, Petnehazi A, Czeller T, Veszelka S, Ormos P, Deli MA, Der A. A versatile lab-on-a-chip tool for modeling biological barriers, 1209-1219.
[12] Lei Z, Jiu-Chao Y, Hana Y, Ning-Xin M, Grace L, Xiangyun AC, Yanming Wa, Li L, Li C, Peng J, Gang-Yi W; Small Molecules Efficiently Reprogram Human Astroglial Cells into Functional Neurons. Cell Stem Cell 2015. DOI: http://dx.doi.org/10.1016/j.stem.2015.09.012
See also :
Blood-brain-barrier content from Nature
Blood-brain-barrier definition from Wikipedia